Understanding Crystalline Types: An In-Depth Exploration
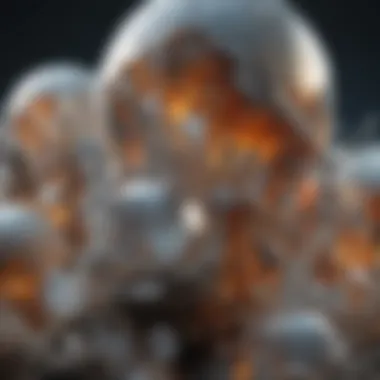
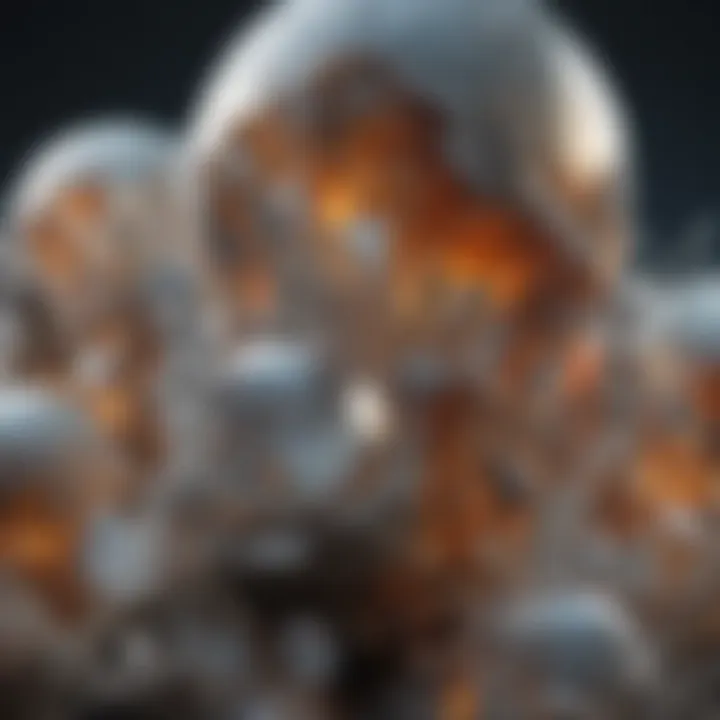
Intro
Crystals are more than just pretty formations. Their unique structures and classifications offer insights into their properties and potential applications. Crystallography, the study of crystals, has broad implications in various fields, including geology, physics, and materials science. Collectors and enthusiasts alike find value in understanding these formations not only for their aesthetic qualities but also for their practical importance. This article seeks to unravel the complexities of crystalline types, shedding light on their characteristics and classification.
Types of Collectibles
The world of collecting is vast. For those interested in crystals, it is essential to grasp the different categories available. Understanding these categories can enrich the collector's experience.
Overview of Collectible Categories
Crystals can be classified into several categories based on various criteria: natural versus synthetic, structural forms, and functional attributes. Hereβs a brief overview:
- Natural Crystals: These are minerals that form over time without human intervention. They are often sought after for their unique appearance and geological significance.
- Synthetic Crystals: Created through human processes, these crystals can mimic the properties of natural ones. Synthetic options are valuable for specific applications, particularly in technology and industry.
- Gemstones: Not all crystals are gemstones. However, gems like diamonds or sapphires offer aesthetic appeal and investment potential.
- Industrial Minerals: These crystals have practical uses in manufacturing and industry. Examples include quartz for electronics and talc for cosmetics.
In-Depth Look at Each Category
Natural Crystals
Natural crystals hold a special allure. They come from the Earth, each with its own unique formation history. Many collectors pursue mesmerizing specimens of quartz or calcite. The rarity of natural occurrences often drives their value.
Synthetic Crystals
On the other hand, synthetic crystals are increasingly in demand. For instance, cubic zirconia is a well-known diamond substitute. These crystals can achieve desired properties faster than nature would allow.
Gemstones
Gemstone collecting is a particular niche. The beauty and durability of gemstones draw in collectors and investors alike. They often require deeper knowledge of grading and valuation.
Industrial Minerals
These minerals tend to be overlooked. Yet, their implications in technology and industry are profound. For example, lithium, found in certain crystalline forms, is crucial for batteries.
Identification Techniques
Identifying crystalline items is crucial for collectors. A strong understanding of identification techniques can help avoid misrepresentation and ensure quality.
Essential Tools for Collectors
Every collector should have some basic tools. Here are a few essential items:
- Loupe: A magnifying lens to see details in crystal structures.
- Hardness Kit: To test the hardness of a crystal based on the Mohs scale.
- pH Test Kit: Useful for identifying certain minerals based on reaction to acids.
Identification Methods for Rocks and Minerals
Different techniques apply to different crystal types. Familiarity with a few standard identification methods can aid greatly in a collectorβs journey:
- Visual Inspection: Observing color, clarity, and form.
- Scratch Test: To determine hardness.
- Acid Test: Identifying calcite and other carbonate minerals.
Understanding the characteristics of crystals is vital for anyone serious about collecting. Knowledge in identification helps ensure that collectors are aware of what they possess.
Prolusion to Crystalline Structures
Crystalline structures play a crucial role in various scientific disciplines, including geology, chemistry, and materials science. Understanding these structures is key to grasping how materials behave under different conditions. In this article, we will explore the different types of crystals, their unique properties, and their significance in both natural and synthetic forms. This overview emphasizes how crystal structures relate to the physical properties of materials, which is essential for applications ranging from industrial utilization to technological innovations.
Defining Crystalline Types
When we talk about crystalline types, we refer to the orderly arrangement of atoms or molecules within a solid. This regular geometric pattern is not only aesthetically intriguing but also determines many physical properties of the material. Crystalline types can be broadly categorized into groups based on bonding interactions, geometry, and symmetry. Examples of these categories include ionic, covalent, metallic, and molecular crystals. Each type exhibits distinct characteristics, influencing how they form, break, or conduct energy.
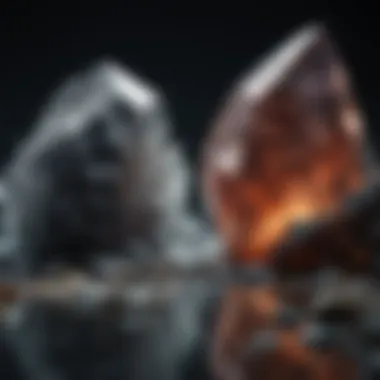
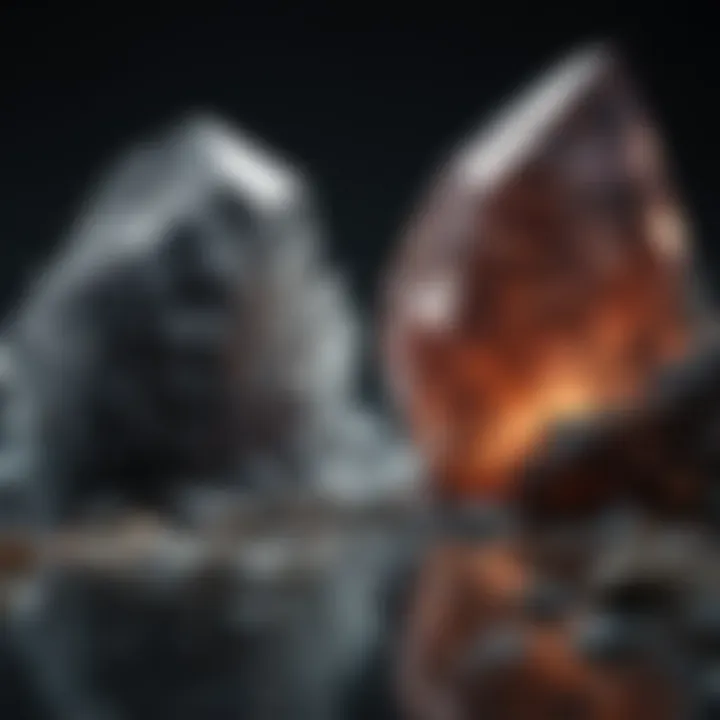
The definition of crystalline types extends beyond just these categories. It is also about understanding how these types interact with the environment and each other. For instance, the ionic bonds in sodium chloride result in a very different crystal structure compared to the covalent bonds in diamond. Such diversity is vital for collectors and industry professionals alike, as it aids in identifying materials and predicting their behavior in various applications.
Historical Context
The study of crystalline structures has a rich history that dates back to ancient civilizations. Early observations of crystals were often based on their visual beauty, leading to the use of gemstones in jewelry. However, the scientific exploration of crystals began to emerge during the 17th century with the advent of crystallography as a field of study. Pioneers like Johannes Kepler and later, RenΓ© Just HaΓΌy, laid foundational work that defined crystal symmetry and structure.
With the development of X-ray diffraction in the early 20th century, the understanding of crystalline structures was revolutionized. This technique allowed scientists to view crystals at the molecular level, leading to significant discoveries in chemistry and materials science. The historical journey of crystallography reflects an ongoing pursuit of knowledge, one that has profound implications not only in our comprehension of natural phenomena but also in the design and development of new materials.
"The arrangement of atoms within a crystal can influence its physical and chemical properties significantly, making the study of crystalline structures essential in various fields."
Today, the ongoing advancements in technology continue to push the boundaries of crystallography, leading to new insights and applications. As we continue to explore crystalline types, understanding this historical context enhances our appreciation for the intricate relationship between structure and function.
Basic Crystal Properties
Understanding the basic properties of crystals is essential for grasping their intricacies. Crystalline structures exhibit unique attributes that influence their behavior and applications in various fields. This section highlights the significance of lattice structures, symmetry, and physical attributes, which together define many critical characteristics of crystals.
Lattice Structures
Lattice structures are foundational to the understanding of crystal properties. The arrangement of atoms in a periodic pattern forms the basis for a crystal's structure, determining its stability and characteristics. The most common types of lattice arrangements are simple cubic, body-centered cubic, and face-centered cubic. Each type affects how crystals interact with light, heat, and other materials.
From a practical viewpoint, the type of lattice structure can affect the physical and chemical properties of the material. For instance, diamonds have a face-centered cubic lattice, which endows them with exceptional hardness and stability. Understanding the lattice structure allows scientists and collectors to predict the behavior of crystals under various conditions.
Symmetry in Crystals
Symmetry plays a crucial role in the classification and identification of crystals. The presence of symmetry in a crystalline solid indicates how the internal arrangement of atoms mirrors itself in various directions. Crystals can possess one or more symmetries, including rotational, reflective, and translational.
This aspect is important not just from a theoretical perspective but also in practical applications. For example, the symmetry of quartz crystals allows them to produce piezoelectric effects, making them highly valuable in electronic devices. Recognizing the symmetry present in different crystals facilitates better identification and classification, aiding collectors and researchers alike.
Physical Attributes
Crystal physical attributes encompass numerous characteristics, including hardness, color, and transparency. These attributes significantly influence the applications and desirability of crystals.
Hardness
The hardness of a crystal, measured on the Mohs scale, indicates its resistance to scratching. This property is pivotal since it determines a crystal's suitability for various uses, such as in jewelry or industry. Diamonds, with a hardness of 10 on the Mohs scale, are highly sought for their durability and aesthetic appeal. On the other hand, softer crystals like gypsum, which scores 2, are less popular in commercial applications due to their fragility.
Hardness not only affects usability but also influences how a crystal can be polished or shaped. Collectors often prefer harder crystals for their durability in display and use.
Color
Color is one of the most visually striking attributes of crystals. The coloration usually arises from the presence of transition metal ions, impurities, or defects within the crystal structure. For example, amethyst is purple due to iron content and exposure to radiation. Different colors can vastly change the value and desirability of crystals among collectors.
Additionally, color can aid in identifying crystals. However, itβs essential to recognize that color can be misleading if not analyzed alongside other properties. Therefore, while color is significant, dependence exclusively on it for identification can lead to errors.
Transparency
Transparency denotes the ability of a crystal to transmit light. This property contributes to how crystals are perceived and valued in the market. Clear crystals, like rock crystal or quartz, are often favored for their optical properties, making them ideal for various applications, from lenses to decorative items.
Nevertheless, not all collectors prioritize transparency. Some prefer opaque crystals for their unique aesthetic features. Understanding the role of transparency helps collectors appreciate the diverse attributes of crystals and can influence their collecting choices.
"The attributes of crystals not only define their physical appeal but also determine their utility across various domains such as technology, medicine, and art."
Thus, grasping the basic properties of crystals provides vital insights into their classifications, applications, and overall significance in both scientific and collecting communities.
Classification of Crystalline Types
The classification of crystalline types holds significant importance in understanding the diverse world of crystals. Each classification elucidates distinct properties, structures, and behaviors of different crystal types, guiding scientists and collectors alike. The systematic categorization aids in the identification and utilization of these crystals across various fields such as geology, materials science, and even technological innovations. Furthermore, by grasping the differences between ionic, covalent, metallic, and molecular crystals, one can appreciate not only their aesthetic value but also their practical applications.
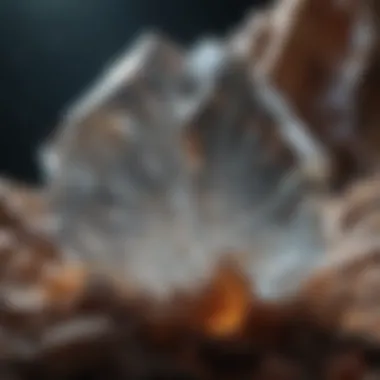
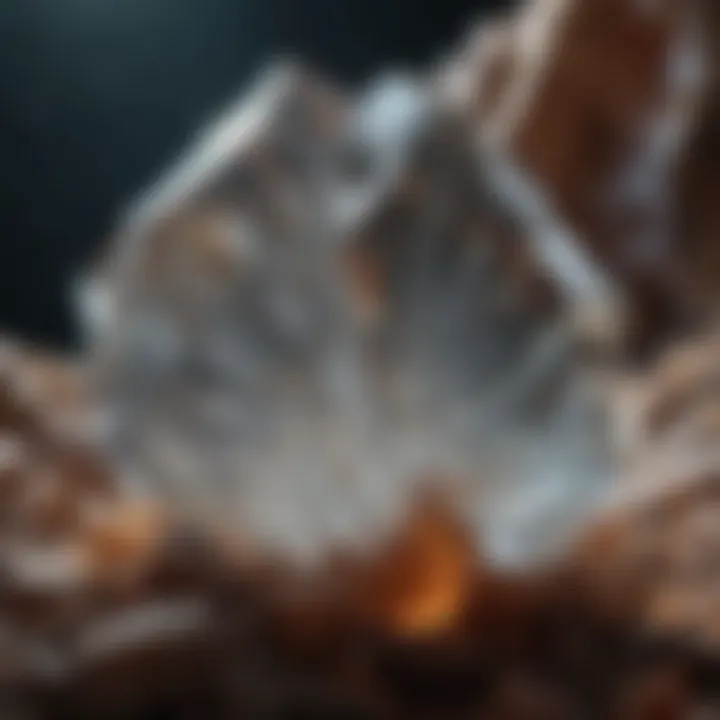
Ionic Crystals
Ionic crystals are formed through the electrostatic attraction between oppositely charged ions. This leads to the creation of a highly ordered arrangement, resulting in solid structures that are usually characterized by their strength and high melting points. Common examples of ionic crystals include table salt, or sodium chloride, and other halides. The ionic bond, being strong, gives these crystals remarkable hardness and insolubility in water.
One distinct feature of ionic crystals is their ability to conduct electricity when molten or dissolved in solution. This property makes ionic crystals essential in various industrial applications, including the production of electrolytes in batteries. The rigid structure of ionic crystals imparts a brittle nature, often leading to shattering when subjected to force.
Covalent Crystals
Covalent crystals arise from atoms sharing electrons, forming a network of bonds that extends throughout the crystal. This type of bonding results in materials that display high melting points and considerable hardness. Diamond is a quintessential example of a covalent crystal, where each carbon atom is tetrahedrally bonded to four other carbon atoms.
The strength of covalent bonds in these crystals lends them exceptional durability and resistance to chemical reactions. As a result, covalent crystals have notable applications in industries such as semiconductor manufacturing, where silicon crystals are vital. Their properties vary significantly from one covalent crystal to another, depending on the nature of the atoms involved in the bonding.
Metallic Crystals
Metallic crystals feature atoms that are held together by a 'sea' of delocalized electrons, allowing for electrical conductivity and malleability. This structure means that metallic crystals can be deformed without breaking, a trait that makes them highly useful in manufacturing. Precious metals, such as gold and silver, exemplify metallic crystals and are prized for both their aesthetic appeal and functional properties.
In terms of applications, metallic crystals are ubiquitous in construction and technology. They serve as fundamental materials in electronics, ensuring efficient conductivity in wiring. The unique properties of metals encompass a variety of behaviors including thermal conductivity, which makes them essential in applications ranging from heat sinks to cookware.
Molecular Crystals
Molecular crystals are formed from molecules held together by weaker van der Waals forces rather than covalent or ionic bonds. This results in a more delicate structure, leading to lower melting points and often more intricate forms. Common substances such as sugar and ice are examples of molecular crystals. Their relatively soft nature allows for easier breakage and manipulation compared to more rigid crystal types.
Despite their generally lower strength, molecular crystals have valuable applications. They often find uses in the pharmaceutical industry for drug formulation, where the purity and crystal form can influence drug efficacy. Moreover, their interesting optical properties make them essential in certain display technologies.
Natural vs. Synthetic Crystals
The distinction between natural and synthetic crystals is significant in understanding crystallography. Each type offers unique benefits and raises important considerations for collectors and researchers. Natural crystals form through geological processes over time. In contrast, synthetic crystals are produced through human-engineered methods in laboratories. These differences impact not only their chemical properties but also their value and applications.
Formation Processes
Natural Processes
Natural crystal formation occurs over millions of years, shaped by environmental factors such as temperature, pressure, and chemistry. The solubility of minerals and their concentration in a given environment play a vital role in this process. The unique characteristic of natural crystals is their intrinsic imperfections, which often enhance their beauty. Natural crystals like quartz or amethyst are appreciated for their aesthetic value and historical significance. However, these imperfections can sometimes make them less desirable for specific industrial uses.
Natural crystals provide authenticity and an intrinsic connection to the Earth. Collectors often seek them for their rarity and geological heritage. However, they can be significantly more expensive depending on their size and quality. The geological conditions that produce natural crystals are sometimes unpredictable, making supply inconsistent.
Synthetic Techniques
Synthetic crystal formation involves controlled processes in laboratories. Techniques such as the Czochralski method or hydrothermal synthesis allow precise control over conditions, resulting in high-purity crystals. A key characteristic of synthetic crystals is their uniformity. They can be produced in large quantities with specific qualities tailored to their intended use, whether in electronics, optics, or jewelry.
Synthetic techniques lead to lower costs and greater availability. Collectors and industries benefit from consistent quality and stability. Synthetic diamonds, for instance, offer similar optical and chemical properties to their natural counterparts but often at a fraction of the price. Yet, some purists argue that synthetic crystals lack the ethereal charm and history of natural ones, which might deter certain buyers.
Applications and Uses
Both natural and synthetic crystals play essential roles in various fields. Natural crystals are often used in jewelry and as decorative items. Their geological stories attract enthusiasts and collectors. On the other hand, synthetic crystals have a broad range of applications in technology. They are crucial in the production of semiconductors, lasers, and fiber optics.
In summary, the choice between natural and synthetic crystals hinges on the intended application and personal preference. Both categories present unique features and advantages that appeal to different audiences.
Practical Applications of Crystalline Types
The practical applications of crystalline types are diverse and far-reaching. Their unique properties influence numerous fields, from industry to medicine. Understanding these applications enables collectors and enthusiasts to appreciate the true value of different crystals. Crystalline materials are not just ornamental; they serve functional roles that underscore scientific principles and innovation.
Industrial Applications
In industry, crystalline materials play essential roles in manufacturing processes. A common example is quartz, which is widely used in electronics for its piezoelectric properties. This property allows quartz crystals to convert mechanical energy into electrical energy, making them vital in oscillators and frequency stabilizers. Metallica crystals like copper, nickel, and lead are fundamental in creating electrical components due to their electrical conductivity.
Moreover, the use of crystalline materials extends to the construction sector. Gypsum crystals are utilized in drywall production, providing essential structural integrity. Cement includes crystalline components that enhance strength and durability, showcasing their significance in construction practices.
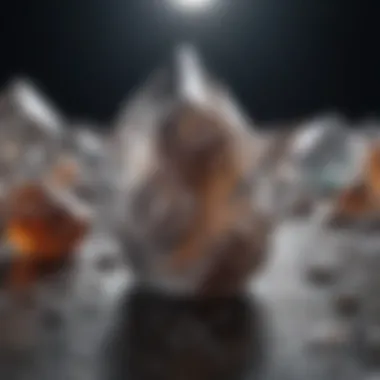
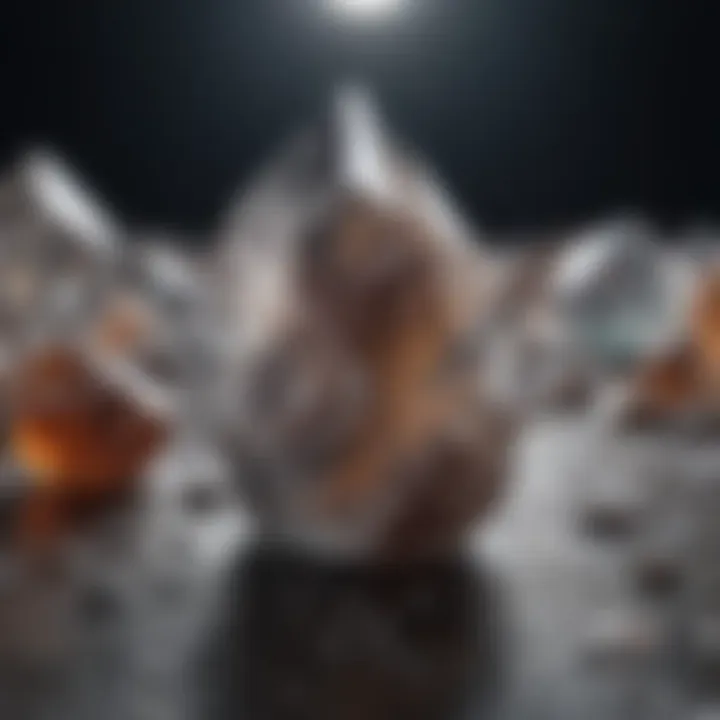
Other critical industrial applications include:
- Optical crystals such as sapphire are used in lenses and cutting tools due to their hardness and clarity.
- Salt crystals (sodium chloride) are essential for food preservation and industrial applications, including chemical production.
- Silicon crystals form the backbone of the semiconductor industry, essential for microelectronics.
Technological Innovations
Technological advancements heavily rely on crystalline materials. One key example is the development of lithium-ion batteries, which are essential for powering modern devices. The cathodes and anodes used in these batteries often involve crystalline structures that optimize ion movement, enhancing battery efficiency.
Another area of innovation is in photonic crystals. These structures manipulate light and have applications in designing advanced optics. They can be used in lasers, optical fibers, and sensors, driving forward communication technologies.
As technology evolves, researchers are exploring more sustainable solutions. For instance, more efficient solar panels utilize crystalline silicon, providing a green energy alternative. This ongoing development in technology shows how critical crystalline materials are in improving efficiency across various domains.
Medical Uses
Crystalline types also find significant applications in medicine. Many pharmaceuticals utilize crystalline forms for better stability and solubility. The purity of crystals often determines the efficacy of medicinal compounds, making their classification a matter of care.
Biologically active crystals, such as calcium phosphate, are used in bone grafting procedures due to their biocompatibility. Another example includes hydroxyapatite, a crystalline form of calcium that is essential for bone health and repair. Understanding the properties of these crystals aids in their application in medical treatments.
Additionally, the field of diagnostics benefits from crystalline types. For example, crystals form in various chemical tests, helping determine the presence of substances in the body. The insights derived from analyzing these types can lead to faster diagnoses and better patient outcomes.
"Crystalline materials hold the key to many sectors, ensuring progress and innovation across fields."
In summary, crystalline types are integral to industrial applications, technological innovations, and medical uses. Their properties and functions enhance various processes, suggesting that a deeper understanding can provide collectors and investors with valuable insights into their significance.
Crystals in Collecting
Collecting crystals can be a rewarding and enlightening pursuit. It encompasses not only the joy of acquiring beautiful pieces but also the vast knowledge one can gain about mineralogy, geology, and the art of identifying unique specimens. For many, it becomes a passion, leading to deeper investigations of crystal formations and their geological stories. This section will explore some essential aspects of collecting crystals, including effective collecting tips and techniques for identifying diverse types.
Collecting Tips
Successful crystal collecting requires careful planning and consideration. Here are key points to enhance the collecting experience:
- Research Locations: Familiarize yourself with local sites known for crystal formation. Certain areas, like the Crystal Caves in Mexico, provide unique opportunities. Exploring online forums can lead to useful information on where to find specific crystals.
- Proper Equipment: Use necessary tools such as a hammer, chisels, and safety gear. A good collection bag is essential for transporting your finds without damage.
- Respect Nature: Always gather crystals responsibly. Ensure you have permission if collecting from private land. It's vital to adhere to regulations to preserve natural landscapes.
- Documentation: Keeping a record of your finds can be valuable. Note the location, date, and conditions under which you found each specimen. This can help you track your collecting journey and learn along the way.
- Join Collecting Clubs: Engaging with like-minded enthusiasts can enhance your experience. Clubs or online groups often host events for swapping, sharing knowledge, and showcasing finds.
Identification Techniques
Identifying crystals can be perplexing given the variety present in nature. Here are several techniques to help:
- Visual Observation: Start with the color, shape, and size of a crystal. These visible traits are often the first indicator of what type you are dealing with.
- Hardness Test: Use the Mohs scale to gauge hardness. Scratching the crystal with materials of known hardness can determine its place on the scale.
- Cleavage and Fracture: Examine how the crystal breaks. Cleavage refers to how well a crystal splits along certain planes, while fracture describes the irregular breakage. Understanding these properties helps narrow down the possibilities.
- Luster Examination: Assess the way light interacts with the crystal's surface. Luster can range from dull to vitreous or metallic, which significantly aids in classification.
"Utilizing a systematic approach to identification can simplify the complexities of recognizing crystalline types and enhance your collecting process."
By applying these tips and techniques, collectors can navigate the world of crystals with confidence and appreciation. Every piece is not just a beautiful object but a window into the history and formation of the Earth itself.
Culmination and Future Directions
This section serves as a pivotal point in understanding the implications and potential future of crystallography. The study of crystalline types is not static; it evolves as new discoveries and technologies emerge. Acknowledging the relevance of this topic extends beyond mere academic interest; it influences various fields such as materials science, geology, and even biotechnology.
Importance of Continued Research
The significance of exploring crystalline types lies in their role in both practical applications and theoretical advancements. From designing stronger building materials to understanding the mechanisms of mineral formation, ongoing research can yield benefits across multiple domains. Emerging technologies, like artificial intelligence, are setting a new pace for discovery. These advancements facilitate more efficient identification and analysis of crystal forms, leading to innovations that were previously thought impossible.
The advancement in crystallography is crucial to innovations in fields like pharmaceuticals, where understanding crystal structures can dramatically affect drug efficacy.
Emerging Trends in Crystallography
Crystallography is experiencing exciting developments. One current trend is the intersection of crystallography with computational models and machine learning. These technologies allow scientists to predict crystal structures and their properties with remarkable accuracy. This predictive capability accelerates the process of crystal design, paving the way for new materials with tailored functionalities.
In addition to computational advancements, there is a growing focus on using crystals for sustainable technology applications. Researchers are developing ways to utilize crystalline materials in solar panels and energy storage devices, showcasing the versatility of these structures. Moreover, the study of biominerals is gaining traction, as understanding how organisms form crystals assists in both health and environmental fields.
Despite these advancements, many challenges persist. There are pressing questions regarding the stability and scalability of synthetic crystals compared to their natural counterparts. Addressing these questions will require collaborative efforts across disciplines, influencing future directions in crystallography.
In summary, the conclusion underscores the vital nature and future relevance of crystalline studies. It is essential to support continuous exploration, which can enhance our understanding of the world while driving innovation in various sectors. As we look ahead, the implications of current research and trends will undoubtedly shape the future landscape of crystallography.