Understanding the Chemical Composition of Graphite
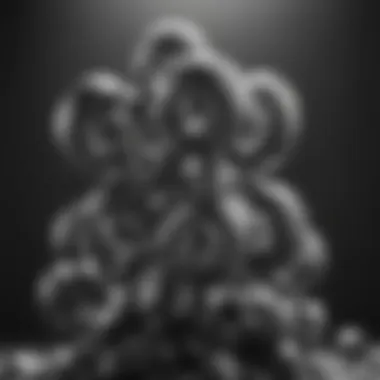
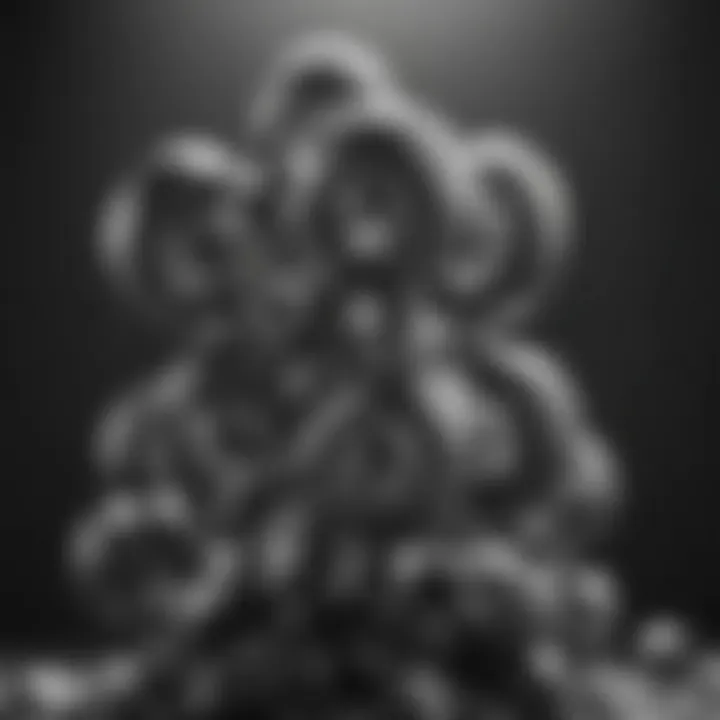
Intro
Graphite, a form of carbon, plays a vital role in numerous scientific and industrial applications. Its unique properties enable it to navigate various fields, notably materials science and geology. With a structure characterized by layers of carbon atoms arranged in a hexagonal lattice, understanding its chemical composition is essential for appreciating its significance. This article delves into the intricacies of graphite's elemental makeup. It aims to equip rock and fossil collectors with insights into this remarkable substance, shedding light on its multifaceted uses and relevance.
Types of Collectibles
When it comes to collectibles, graphite might not immediately spring to mind. However, its unique forms and variations make it intriguing for collectors. Below, we explore the categories of graphite that enthusiasts often seek out.
Overview of Collectible Categories
There are two main categories of graphite collectibles:
- Natural Graphite: This includes varieties found in nature, such as flake graphite, lump graphite, and amorphous graphite. Each type has a different structure and appearance, which can affect their desirability among collectors.
- Synthetic Graphite: Generally produced through industrial processes, synthetic graphite mimics natural types and often has specific attributes that cater to modern applications, like batteries or lubricants. Collectors with a scientific bent might find synthetic varieties particularly interesting.
In-Depth Look at Each Category
- Natural Graphite
- Synthetic Graphite
- Flake Graphite: Known for its crystalline structure, flake graphite is often moderated when used in industrial applications but attracts collectors thanks to its unique geometric patterns.
- Lump Graphite: This type appears as large, often lustrous pieces. It can be found in various locations globally, making it an exciting find.
- Amorphous Graphite: With a less-defined structure, amorphous graphite tends to have a duller appearance, yet it intrigues collectors due to its rare formation process.
- Electrode Graphite: Precisely engineered for performance, this type is one of the most crucial in electrical applications. Collectors may focus on its distinct production history.
- Expanded Graphite: Produced through the exfoliation of natural graphite flakes, expanded graphite has unique properties and applications, appealing to those interested in modern materials science.
Identification Techniques
Understanding how to identify different types of graphite can greatly enhance a collector's experience. Here are some essential tools and methods to consider.
Essential Tools for Collectors
- Hand Lens: A simple yet effective tool, a hand lens helps examine graphite in detail, allowing collectors to observe its structure and layering.
- Mohs Hardness Test Kit: This kit can determine the hardness of the graphite sample, giving insight into its type.
- Field Guide: Having a reference guide to assist in identification can be a valuable asset during expeditions.
Identification Methods for Rocks and Minerals
- Visual Inspection: Begin by closely observing the color, sheen, and crystal structure of the graphite. Each type often has distinct characteristics.
- Scratch Test: Utilize a fingernail or penknife to perform the scratch test. The resistance against scratching can provide clues about the graphite’s identity.
- Water Test: Dropping a small amount of water can help evaluate the hydrophobicity of the sample, which varies among different types of graphite.
"Graphite is not just a mere pencil material, it's a bridge connecting natural wonders and industrial marvels."
The unique characteristics of graphite extend beyond visual appeal. Its chemical composition—intriguingly complex—underpins its properties and applications in modern society. For rock and fossil collectors looking to appreciate more than just the surface, understanding these nuances can elevate their collection to new heights.
Foreword to Graphite
Graphite is more than just a simple carbon allotrope; it's quite an extraordinary material, pivotal in a myriad of industries ranging from electronics to metallurgy. Understanding graphite is essential for anyone venturing into geology, materials science or perhaps, even rock collection. With a unique set of properties and varied applications, graphite's significance cannot be understated.
As this article unfolds, we will delve into multiple facets of graphite, particularly focusing on its chemical composition. We’ll explore how the arrangement of carbon atoms impacts its performance in different roles, as well as its historical journey from a mere carbon variant to a cornerstone of modern technology.
Defining Graphite
Graphite, fundamentally composed of carbon, is recognized for its unique structure. It occurs naturally, presenting itself in various forms like flaky, amorphous, and lump varieties. Each form exhibits distinct characteristics, dictated by its formation conditions and the arrangement of its atomic layers.
The carbon atoms in graphite are arranged in sheets, linked together by weak Van der Waals forces. This layered structure not only grants graphite its characteristic lubricating properties but also allows for easy electrical conductivity, making it a key player in numerous applications. Thus, when we define graphite, we are looking at a material that is not only versatile but also inherently complex due to its atomic architecture.
Historical Context
The story of graphite is wrapped in ages and cultural significance. First discovered in the 16th century in Borrowdale, England, graphite was initially regarded as a curious mineral. The first uses were rather rudimentary: locals found it effective for marking sheep.
"Graphite's true potential was gradually recognized and leveraged, transforming it from a pastoral tool to a resource invaluable for technological advancement."
By the 19th century, it became clear that graphite was indispensable for not just writing instruments but also for various industrial applications such as lubricants and batteries. Throughout history, its utility evolved, paving the way for its crucial involvement in modern technologies, marking a significant leap from its simple roots.
In summary, this microscopic journey through the life of graphite illustrates not just its definition and history but sets the stage for a deeper examination into its chemical composition. Understanding this element's past and present empowers collectors and enthusiasts alike to appreciate its importance in today’s technological landscape.
Basic Chemical Composition
Understanding the basic chemical composition of graphite is crucial for anyone delving into its multifaceted nature. Not only does this anchor the discussions of its properties, but it also lays the groundwork for various applications in scientific, industrial, and even artistic realms. Graphite represents a fascinating case study of how elemental makeup shapes a material's characteristics and potential uses.
Elemental Analysis
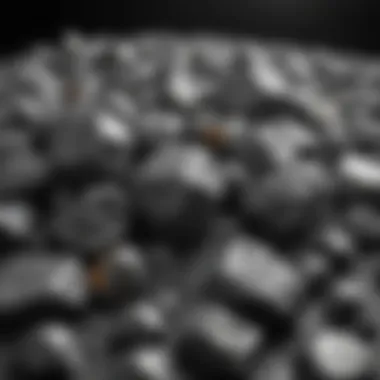
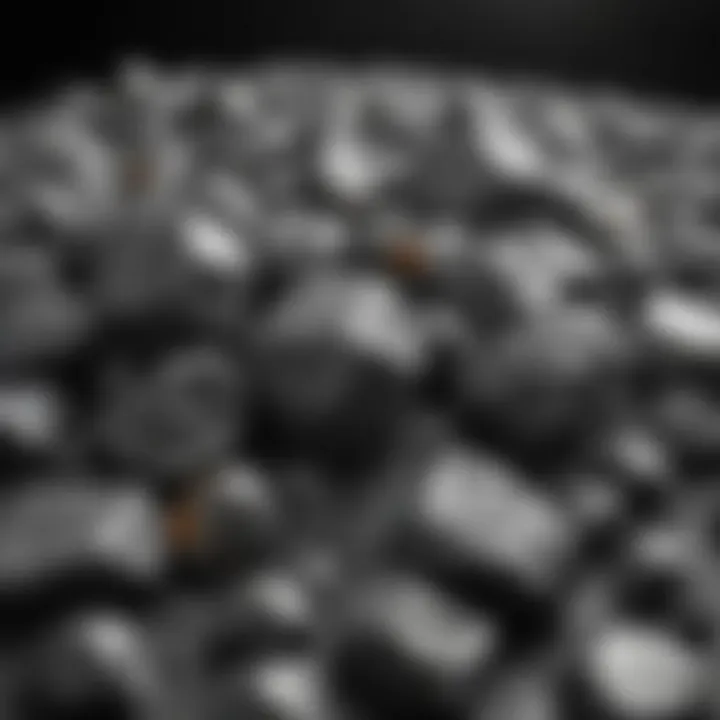
When we consider the elemental analysis of graphite, the spotlight is firmly on carbon—what else? Graphite is made nearly entirely of carbon atoms, typically presenting a content that hovers around 90% to over 99%. This high purity is what makes graphite so desirable in numerous applications, ranging from batteries to writing implements.
To break it down:
- Carbon: The dominant element, forming the structure of graphite.
- Other elements: Depending on the source, traces of sulfur, hydrogen, and oxygen might also be present. These impurities can influence the material’s characteristics, such as conductivity and reactivity.
A notable feature of carbon in graphite is its ability to bond with itself in a unique hexagonal lattice arrangement. This arrangement ensures that layers of carbon atoms can easily slide over one another, which is what gives graphite its lubricating properties as well as its classic writable qualities.
Carbon Content and Purity
The carbon content in graphite directly associates with its purity and overall quality. High carbon purity is often equated with better performance, especially in applications requiring thermal and electrical conductivity. When considering graphite for high-tech applications, even tiny impurities can impact performance.
Several grading systems exist, distinguishing between different purities of graphite:
- Natural Graphite: Often contains impurities such as silica or iron, which can lower its conductivity.
- Synthetic Graphite: Purified and often engineered to achieve very high carbon content. Its applications see it used in advanced batteries and other technologies.
Therefore, when searching for the right type of graphite, one must always consider carbon content and purity. Whether you dabble in collecting or are a seasoned rock hound, knowing the specific carbon content can help you comprehend the potential value and usage of your graphite specimens.
"The unique carbon content of graphite makes it essential in many technological applications while also presenting an exciting avenue for collectors to explore."
Crystalline Structure of Graphite
The crystalline structure of graphite is not just a dry subject of study; it’s the backbone of its unique qualities and plays a pivotal role in how this mineral is utilized across various fields. More than just a collection of carbon atoms, graphite exhibits a well-organized layered arrangement that fundamentally shapes its physical properties and functionality. Understanding this structure opens the door to appreciating graphite’s applications, from high-tech industries to everyday items, particularly for enthusiasts and collectors looking for deeper insights into this fascinating material.
Layered Arrangement
Graphite’s crystalline structure features a remarkable layered arrangement. Each layer is held together by weak van der Waals forces, which allow them to glide over one another with ease. This unique configuration is responsible for graphite’s lubricating properties and its use in pencils, as these layers can easily shed from the bulk material.
Furthermore, the spacing between these layers can influence the material's electrical conductivity. The ability to delaminate—or separate—these layers makes graphite not just a pretty face in mining but a heavy hitter in various applications like batteries and superconductors.
"In graphite, the carbon atoms are arranged in a 2D honeycomb lattice. This unique formation creates a material that is both strong and conductive, leading to a world of possibilities."
In general, there are a few noteworthy characteristics of this layered arrangement:
- Orientation: The orientation of the layers can impact the mechanical strength and electrical properties. For instance, the electrical conductive properties can vary based on whether the measurement is taken parallel or perpendicular to the layers.
- Layer Thickness: The number of layers can give graphite a range of properties. Few-layered graphite exhibits greater conductivity compared to bulk graphite, drawing more attention from industries focused on advanced materials.
- Interlayer Interactions: As layers can interact, understanding these interactions may lead to enhancing properties such as thermal management or improving the efficiency of energy storage devices.
Bonding Characteristics
When delving into graphite's crystallinity, bonding characteristics cannot be overlooked. The carbon atoms within each layer are bonded through strong covalent bonds (sp² hybridization), forming a flat structure that is highly stable. Yet, amid this stability lies flexibility, since these covalent bonds within the layers contrast sharply with the weak van der Waals forces that govern the interaction between layers.
The implications of these bonding characteristics are profound:
- Conductivity: The sp² hybridization facilitates electrical conductivity because the delocalized π-electrons move freely within the planes, making graphite a conductor of electricity. This is one reason why graphite finds its way into an array of electronic applications, from batteries to novel materials.
- Chemical Reactivity: The nature of bonding impacts how graphite interacts with other elements and compounds. For instance, when reacting with oxidizing agents, graphite can demonstrate different behaviors depending on the conditions and the layer's exposure.
- Material Strength: These bonding characteristics also contribute to its impressive mechanical strength, making graphite useful in demanding applications, such as aerospace components and sporting goods.
Ultimately, the structural and bonding arrangements of graphite are foundational to its myriad of uses. From high-performance batteries to simple lubricants, understanding how these characteristics interplay can provide rock and fossil collectors with a richer appreciation of not only graphite itself, but also the broader gallery of materials that rely on it.
Variations in Composition
Understanding the variations in composition of graphite is like peeling an onion. Each layer reveals unique properties that can profoundly influence its applications and characteristics. The differences can stem from natural formations or methods of synthetic production. By examining these variations, we gain insight not just into the material itself, but also how it interacts with various technologies and processes in science.
Natural vs. Synthetic Graphite
Natural graphite is a gift from Mother Nature, extracted from metamorphic rocks, mainly formed over millions of years under heat and pressure. The most common sources include flake graphite, found in veins, and amorphous graphite, which has a more random structure. These natural forms exhibit unique traits, such as varying degrees of crystallinity and purity, affecting their functionality in applications like lubricants or batteries.
In contrast, synthetic graphite hails from human ingenuity, primarily produced through high-temperature processes that convert carbon materials like petroleum coke or coal tar pitch into graphite. The controlled environment during its formation means we often achieve a more uniform quality and higher purity level. This synthetic variant proves invaluable in scenarios demanding high performance, such as in electrodes for electric arc furnaces or in lithium-ion batteries. Importantly, the choice between natural and synthetic graphite isn't merely a question of cost; it's about the specific demands of the project at hand, balancing purity and performance with availability and cost-efficiency.
"The choice between natural and synthetic graphite can significantly affect the performance of final applications, so understanding their differences is crucial for any collector or enthusiast."
Isotopic Composition
Delving into isotopic composition provides yet another layer of complexity and interest. Graphite primarily consists of carbon-12, the most prevalent isotope, but variations can exist, especially in natural graphite. Carbon-13, though less common, is particularly useful in fields like geology and ecology because it can reveal information about the sources and conditions of formation. This isotopic variation can be traced back to geological processes, making it a valuable tool for researchers looking to understand the environmental conditions that affected carbon cycles.
Isotopic analysis isn’t limited to field studies; it’s also a key player in understanding the behavior of graphite in material science. By studying isotopes, scientists can simulate and predict the performance of graphite in industrial applications, leading to innovations in product designs and enhancements in technology. Collectors interested in the origin stories behind their specimens will find insights in isotopic variations, possibly elevating their appreciation for the pieces in their collections.
In summary, whether choosing between natural and synthetic graphite or exploring the implications of isotopic differences, understanding variations in composition reveals critical insights that influence both practical applications and the scientific curiosities of graphite, enriching our engagement with this multifaceted material.
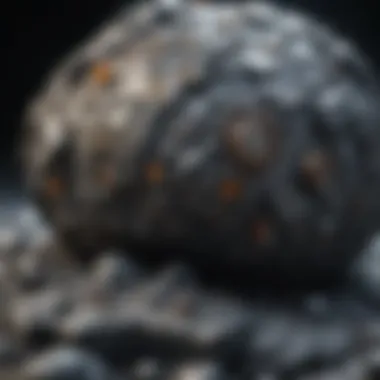
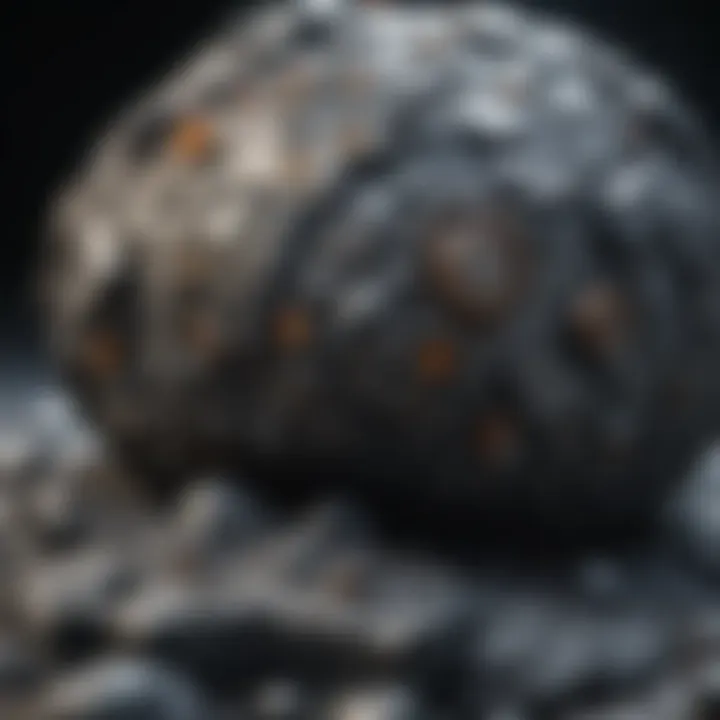
Graphite's Properties and Applications
Understanding the properties and applications of graphite is essential for a myriad of industries and scientific fields. Graphite isn't just a mere carbon allotrope; it possesses unique properties that make it incredibly versatile. The interplay between its molecular structure and the inherent characteristics it displays paves the way for numerous innovative applications. For instance, its electrical and thermal conductivity set it apart in technology-driven sectors, while its mechanical properties ensure that it remains a staple material in construction and manufacturing. As we dive deeper into its electrical behavior, thermal capacities, and mechanical strength, it becomes clear just how pivotal graphite is in both natural and synthetic contexts.
Electrical Conductivity
Electrical conductivity is one of graphite's crowning attributes. The structure of graphite facilitates the movement of electrons freely along its planes, which is paramount for its function as a conductor. Unlike metals, which rely on a sea of electrons to transfer electricity, graphite's unique layered arrangement allows electrons to flow smoothly through the layers. This property has led to its application in various electronic components such as batteries, electrodes, and even some types of transistors.
- Key Advantages:
- High conductivity makes it ideal for use in batteries like lithium-ion.
- Stable performance, even under varied temperatures, lends durability.
- Lightweight nature contributes to portability in electronic devices.
Investments in graphite for these applications have surged with the rise of electric vehicles and renewable energy technologies, reinforcing its position as a critical material in modern advancements.
Thermal Conductivity
Following up on electrical performance, thermal conductivity in graphite is another trait worth noting. Its ability to efficiently dissipate heat is invaluable in applications ranging from industrial machinery to consumer electronics. This characteristic results from the same layered structure that benefits its electrical conductivity, allowing heat to transfer effectively between the planes.
- Applications in Various Sectors:
- Used in heat sinks for electronics, reducing overheating and ensuring longevity.
- Essential for manufacturing refractory materials, contributing to high-temperature furnaces.
- Plays a role in batteries, where heat management is critical to efficiency and safety.
Due to these factors, graphite has earned its place in the production of parts that must withstand extreme conditions, proving itself to be resilient and reliable.
Mechanical Properties
When it comes to mechanical properties, graphite showcases a blend of strength and flexibility that is tough to beat. Its layered structure means that although it may be soft, it can withstand significant pressure without breaking apart. This unique combination allows graphite to be molded into various forms, such as lubricants, composites, and structural components.
- Versatile Use Cases:
- Its lubricating properties make it ideal for high-temperature applications where other lubricants fail.
- In composite materials, it enhances strength without adding excessive weight.
- Graphite's resilience is harnessed in pencil lead, where a balance of softness and strength is crucial.
Graphite's mechanical features not only make it a preferred choice for many engineering needs but also open pathways to new material research and application realms.
The ability of graphite to combine strength, flexibility, and thermal efficiency makes it a go-to material in both everyday items and advanced engineering products.
In summary, the properties of graphite—particularly its electrical and thermal conductivity, along with its mechanical strengths—paint a picture of an element that is not only foundational in our current technology landscape but also full of potential for future innovations. Understanding these traits allows enthusiasts, collectors, and industry professionals alike to appreciate just how essential graphite is to modern life.
Graphite in Nature
Graphite is more than just a pencil lead or industrial material; it is a natural phenomenon that contributes significantly to our Earth’s geology and material science. The study of graphite in nature allows us to appreciate its formation processes, locate various deposits, and understand its ecological role.
Occurrence and Formation
Graphite predominantly forms in metamorphic rocks, resulting from the alteration of carbon-containing rocks like limestone or organic materials under high pressure and temperature. This transformation creates crystalline structures that we recognize as graphite. Depending on its geological setting, graphite can be categorized into various types like natural flake graphite, amorphous graphite, and lump graphite. In metamorphosed deposits, you might find flaky or crystalline graphite, while sedimentary environments are more likely to yield amorphous varieties.
Graphite can also occur in volcanic and hydrothermal environments, where certain conditions lead to its precipitation from mineral-rich solutions, contributing to unique deposits that may retail interesting properties for future applications.
- Rich Sources of Natural Graphite:
- Graphitic Schists: This type of rock metamorphism produces large quantities of graphite.
- Igneous Systems: In some cases, granite and pegmatites serve as host rocks for graphite formation.
Understanding the occurrence of graphite helps collectors and enthusiasts locate valuable deposits. Notable locations around the globe include Madagascar, China, and Canada, each offering distinct forms and qualities worth exploring. As rocks tell stories of ancient processes, the presence of graphite within them whispers of high-pressure environments, rich organic materials, and time itself.
Geological Significance
Graphite plays a vital role in geology, particularly in understanding Earth's history and geological processes. It serves as an indicator of metamorphic conditions, helping geologists decipher the thermal and pressure regimes that affected its formation. The mineralogy of graphite can reveal much about the past geological events, such as continental drift, volcanic activities, and the evolution of organic life.
Moreover, graphite deposits can have economic implications. They represent categories of ores that can be mined for various industrial, technological, and even artistic applications. When investigating graphite within geological studies, some significant aspects to consider are:
- Metamorphic Geological History
The presence of graphite often indicates a history of high-grade metamorphism in a region, marking areas of significant geological activity. - Resource Valuation
The concentration and quality of graphite deposits can influence local economies, creating avenues for mining and trade. - Environmental Impact
As graphite is extracted, attention must go to the preservation of surrounding ecosystems, necessitating a balance between resource utilization and environmental stewardship.
Graphite thus serves not only as a mineral of interest but also as a beacon of the stories embedded deep within our planet. In recognizing its geological significance, collectors can appreciate the narratives these materials carry, while also considering the implications for the environment and society as a whole.
"Graphite is not just a component of pencils, it is a matrix of history written in the language of geology, waiting for explorers and scholars alike to read its tale."
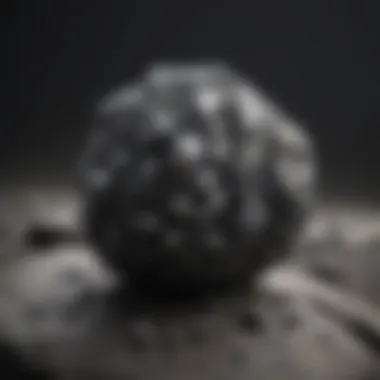
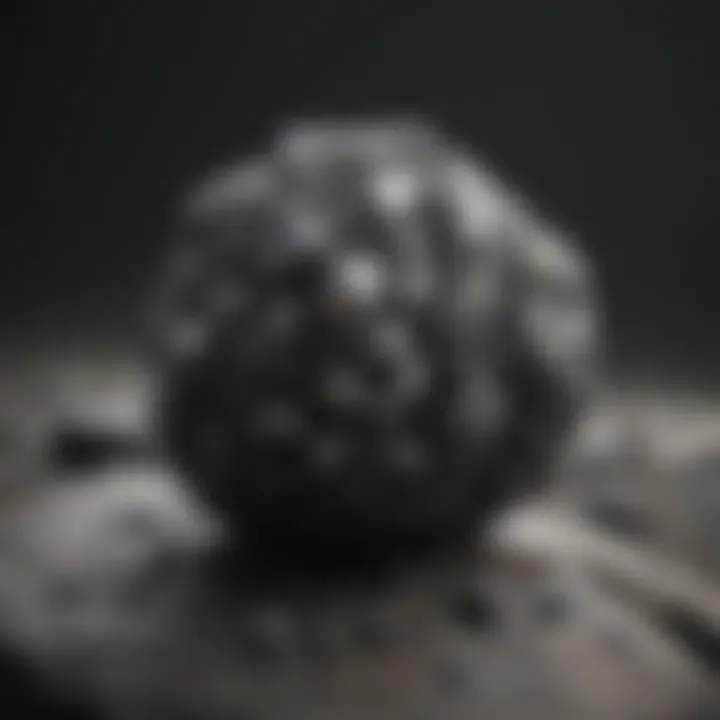
Extraction and Processing
The extraction and processing of graphite are pivotal topics within the study of its chemical composition. Understanding how graphite is gathered and refined underscores its significance in various industries, as well as the environmental implications associated with its extraction. As the demand for graphite rises, particularly in the fields of technology and energy storage, the methods of obtaining and purifying this material warrant close examination.
Mining Techniques
Mining graphite involves techniques that vary depending on whether the material is found as natural flake graphite or as amorphous graphite.
Natural flake graphite is usually mined using conventional open-pit methods. This technique involves removing layers of soil and rock to expose the graphite deposits beneath. In contrast, amorphous graphite is typically mined using underground techniques due to its location in veins deep within the earth.
The choice of mining method can significantly affect the quality and purity of the graphite obtained.
- Flake graphite usually contains higher carbon content and fewer impurities compared to amorphous graphite.
- Notably, the quality of the extraction affects subsequent processing steps, thereby influencing the material's performance in applications such as batteries and lubricants.
In recent years, environmental concerns have prompted some companies to explore more sustainable mining practices. Such practices might include:
- Reducing water usage during extraction.
- Implementing land reclamation efforts post-mining.
- Minimizing the carbon footprint of transportation routes.
Each technique has its unique challenges as well, often requiring careful planning and management to ensure that the ecological impact remains minimal while meeting industrial needs.
Purification Methods
The purification of graphite is just as critical as its extraction. After mining, the raw graphite often contains impurities that can affect its performance in various applications. The goal of purification is to enhance graphite's carbon content and ensure its suitability for specific uses.
Several methods exist for purifying graphite. One common approach is flotation, which uses chemicals to separate graphite from other materials based on differences in their surface properties. The flotation process usually yields high purity graphite, ideal for high-tech applications. However, the chemicals used must be handled with care to avoid environmental contamination.
Another method, called thermal purification, utilizes high temperatures to burn off impurities adhering to the graphite. This technique is particularly effective at eliminating volatile compounds.
A common challenge arises when dealing with various grades of graphite. Some high-grade grades need less purification, while others, particularly flake graphite, must undergo extensive purification to remove any foreign material that may lower its effectiveness in applications such as batteries.
Purification not only enhances the quality of graphite but also opens new avenues for its uses across modern technologies.
Future of Graphite Research
The landscape of graphite research is dynamic and evolving. As industries push for new materials that can withstand the demands of modern technology, understanding the chemical composition of graphite becomes increasingly vital. This section delves into innovative applications and essential environmental considerations, two elements that highlight the potential future paths for graphite research.
Innovative Applications
Graphite is staking its claim in numerous fields that traverse traditional boundaries. One of the most exciting areas is energy storage. Lithium-ion batteries, which power everything from smartphones to electric vehicles, rely heavily on high-quality graphite for their anodes. As researchers work hand-in-hand with manufacturers, they are constantly looking for ways to enhance the efficiency and lifespan of these batteries. The push for higher-capacity batteries demands a deep dive into graphite's structural properties, pushing the envelope in both sourcing and processing methods.
Apart from batteries, graphene — a single layer of graphite — has taken the spotlight in materials science. The potential of graphene in electronics, medical devices, and even water filtration systems cannot be overstated. Researchers are exploring how to synthesize graphene in a cost-effective manner, leveraging its unique properties for better-performing devices.
Moreover, advancements in composite materials are abundant. Sculpting intricate materials from graphite can lead to lightweight yet strong components, which is essential in the aerospace industry. High-performance sports equipment like bicycle frames, also benefit from the incorporation of graphite, showcasing its versatility.
The emerging field of nanotechnology also opens new frontiers. Researchers are investigating how nano-sized graphite particles can enhance performance characteristics in various applications, from coatings to pharmaceuticals. Such tiny systems may yield insights into molecular interactions, leading to the development of more effective therapeutic agents.
Environmental Considerations
In the face of green technology demands, the extraction and processing of graphite implicate various environmental concerns. Mining graphite, particularly when undertaken irresponsibly, can lead to significant ecological degradation. Thus, the graphite research community must address how to balance industrial growth with environmental stewardship.
Efforts to develop more sustainable extraction processes are becoming paramount. Techniques that minimize land disruption and reduce waste are high on the priority list. Innovations such as biomining, which uses bacteria to extract metals from ores, are being applied to graphite mining, presenting a less invasive alternative to traditional methods. This demonstrates a commitment to preserving natural habitats while still meeting industrial demand.
Moreover, recycling graphite from used batteries and other sources is a burgeoning area. As the demand for graphite increases, closing the loop on waste will prevent resource depletion and lessen environmental impact. Innovative recycling processes turn waste into valuable resources, thus contributing positively to the ecosystem.
"The pathway forward for graphite lies in enhanced research that consciously integrates environmental sustainability with technological innovation."
The End
The final section of this exploration encapsulates the chemical composition of graphite as a multifaceted subject, rich with implications and significance for various stakeholders, including rock and fossil collectors. When we look back at the previous discussions, it becomes increasingly clear that understanding graphite’s structure and properties isn’t merely an academic exercise. This knowledge is pivotal, not just for scientists, but for enthusiasts who appreciate the intricate nuances of this remarkable material.
Summarizing Graphite's Value
Graphite’s value extends beyond its chemical makeup. It holds industrial significance due to its exceptional thermal and electrical properties, making it an indispensable resource in numerous applications. For example, its conductivity lends itself well to technologies ranging from advanced batteries to electrodes in electric arc furnaces. The high carbon content offers a unique advantage, presenting opportunities for innovation in energy storage and electronics.
Moreover, graphite is emerging in other fields, like lubriants or even as an ingredient in cosmetics, showcasing its versatility. For rock and fossil collectors, the allure of graphite isn't solely in its utility but also in its aesthetic attributes and geological significance. This makes it an attractive area of focus for personal collections and educational pursuits.
Implications for Collectors and Enthusiasts
The implications of this article for collectors can't be overstated. As the market for specialty minerals and graphitic materials expands, enthusiasts have the opportunity to deepen their understanding of the various types of graphite available, such as flake graphite or amorphous graphite. Understanding the specific chemical properties can help in distinguishing between high-quality specimens and those that might not meet the same standards.
Moreover, collectors can benefit from a keen awareness of the environmental and industrial factors that influence graphite mining and processing today. With growing concerns about sustainability and ethical sourcing, knowledge of how natural graphite is extracted and the methods used for purification can guide responsible collecting.
"Understanding the intricacies behind graphite’s chemical properties can elevate a collector's appreciation for this unique material."
In a world that's evolving, the importance of the subject examined in this article is destined to grow. As innovations continue to unfold, staying informed about the chemical composition and applications of graphite allows collectors to appreciate not only the material's beauty but its significant role in shaping our technological landscape.